Microscope Objective Lenses
Created | Updated Jul 22, 2002
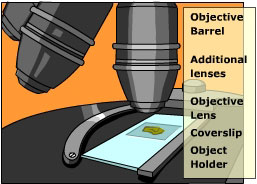
The central piece of an optical microscope is its objective lens. Most objective lenses should strictly be called 'objective lens arrays', since they are made out of many lenses - but no-one calls them that.
The lenses that make up the objective lens array are commonly encased in a metal cylinder a few centimetres in height, and about one centimetre in diameter. Such a cylinder is often termed an 'objective barrel'. At the top and bottom of the barrel are the lenses (and there can be many more lenses arranged in complicated ways in the inner part of an objective barrel). At the top of the barrel there is also a screw with which one can attach the objective lens to the microscope (normally on a revolving objective drum). The bottom end of the barrel will be nearest to the object to be inspected. Specifications are engraved on the side of the cylinder, giving information about the type, the magnification power and the resolution of the objective lens.
An objective lens is very generally defined as the first lens of any optical apparatus from the perspective of the object (hence the name). The term is not confined to microscopy, and can be found in other fields of optical science; this Guide Entry, however, describes the aspects of objective lenses concerning microscopy in particular.
The Objective Lens
In microscopy there are different types of objective lens. Their general appearance is more or less the same; but their magnification can vary, and some special objectives require extra handling and adjustment, while some are more simple. Above is an illustration of a generic microscope objective lens array. In the example shown, the objective lens is a compound of two lenses which work together as if they were just one stronger lens. Many of the microscope objective lenses are compounded of many weaker lenses to form one strong lens.
Magnification, Numeric Aperture and Resolution
Strangely, the magnification factor is the least important property of an objective lens. It's merely equivalent to the strength of the lens array. The bigger the magnification factor, the more the user will be able to zoom in. But the ability to zoom in is of little help if the resolution is low. On the other hand, high resolution makes little sense unless one is able to zoom in.
The resolution is defined by the smallest distance between two dots that still allows them to be recognised as two distinct points. The problem with microscopy is that these dots have a minimum size, and this size depends in its turn on the sharpness of the dots. So the crucial question is: How sharp can images get? Or, the other way round: How blurry do dots get?
The reason for the blurriness lies in the fact that not all of the light from any one point of the sample can be collected. The objective lens has a finite width, and it is placed at a finite distance from the observed dot. Hence only a cone of light can be collected by the objective lens. And it's not the loss of intensity but the loss of directional information that is the trouble. The problem lies in the missing interference pattern at the edge of the projected dot - it gets blurred.
As if that was not enough, the missing interference also depends on the colour of the light. So in the end, the blurriness and thus the resolution depends on the wavelength of the light used, and the width of the collection cone. The distance between the two dots decreases with the cone size (Numeric Aperture) and increases with the wavelength. The rough formula is: wavelength of the light (in hundreds of nanometers) divided by roughly twice the numeric aperture (which is around unity). So - even more roughly - the resolution is about half the wavelength.
Increasing the Numeric Aperture
There is a neat little effect in optics called Total Internal Reflection. This is what makes the surface of a lake or a glass plate look like a mirror at shallow angles. The angle where light starts to get reflected (the Critical Angle) is proportional to the quotient of the refractive indices of the media in question. If this quotient is high (eg going from water to air) then the angles get less shallow, if the quotient is low (eg going from glycerine to glass) then the angles get wider. Now how does this affect numeric aperture?
How can the detection cone get wider? The answer is: When the refractive indices of the medium between the object and the first lens come close. On the other hand, because of this, the magnification power of this lens will diminish. There is an optimal compromise between losing lens power and increasing the numeric aperture. For that reason some objectives work in special immersion media, like special immersion oils or water. These objective lenses have a very complex design to optimise the effect of the immersion medium.
Types of Objective
Some types of objective have already been presented in the preceding section. These use some special working mechanisms, such as immersion, and the so-called 'air' (or non-immersion) objectives, which also vary in magnification factors and numeric apertures.
There are more variations, stemming from the effort to correct certain optical flaws, or aberrations. There are two types of aberration which can be corrected.
Chromatic aberration happens because blue light is bent by a material to a greater extent than red light. To circumvent this, the polychromatic light is sent through a convex lens with a lower refractive index but with the same curvature after each lens. The magnification effect will remain, but the colours will be shifted the other way round, compensating for the aberration. Objectives corrected for this aberration are called Achromatic.
Spherical aberration is due to the fact that most of the lens curvatures are derived from the surface of a sphere1. For this reason, the peripheral area of the lens will focus a bundle of light on a position different from the focal point of light going through the central part of the lens. This aberration is weak for lenses with small curvatures, and more pronounced for stronger lenses. A combination of lenses arranged in very special positions compensates for this aberration. Lenses with such a corrective arrangement are called 'aspheric'. However, aspheric lenses are very sensitive to changes in the medium between the object and the lens, like cover slips with different thicknesses or different optical qualities. This problem can be corrected by re-adjusting the lens positions.
To sum up, there are two main types of objective: 'immersion' objectives and 'air' objectives. These can both be achromatic and/or aspheric. One further exotic type is the 'mirror' objective, which works in the same way as a mirror telescope, but is a lot more difficult to handle. It has the advantage that it doesn't lose as much light-intensity as 'lens' objectives. All of these objectives are available in further, more specialised variations, which allow a finer tuning of optical possibilities.